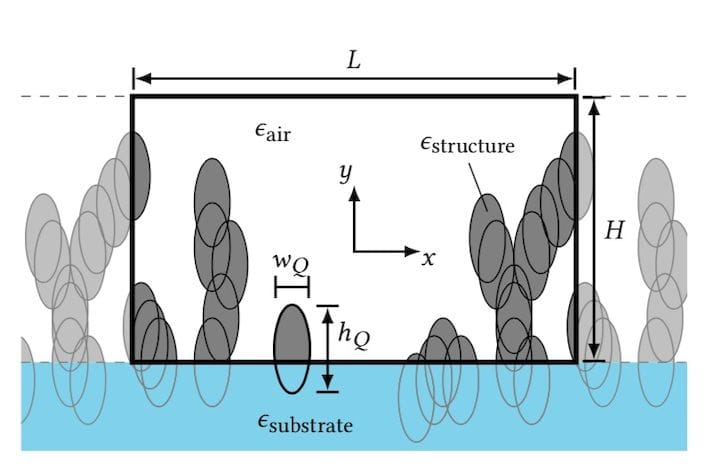
I’m learning a lot more about how light and color actually work after reading a research paper on an experimental method for 3D printing colors.
Color 3D printing is a bit of a rarity these days, with only a few 3D printer manufacturers taking on the challenge. The approaches used by these companies, including Stratasys, Mcor Technologies, Mimaki and a few others, typically involve introducing colored ink into the 3D printing process.
Such approaches usually mix base colors, like RGB or CMYK, to obtain desired colors in the output object.
This approach is how we usually understand how we see color: white light is bounced off an object, but the reflective surface absorbs some colors, and the remaining reflected frequencies are what we see. The classic example is green grass: the vegetation absorbs red and blue frequencies, leaving only the green visible.
This is how paint works. It’s also how inkjets and color 3D printers work. Light is absorbed and the remainder is reflected.
But this is not how the researchers from Austria and Saudi Arabia devised their new approach to implementing 3D printed colors. Instead, they used an approach called “structural colorization”.
Structural colorization is a technique in which a color is generated because the shape of a surface causes only certain frequencies to be reflected. This is different from frequencies being absorbed.
Apparently, and I did not know this, many unusual colors in nature are generated in this way. The researchers explain:
“Many brilliant colors in nature do not arise due to light-absorbing pigmentations but due to interference effects caused by nanostructures. A wide variety of structures, which serve basic functions such as camouflage or courtship, can be observed both in flora and fauna, such as layered structures, 3D photonic crystals, and surface structures.
By understanding these shapes and geometries, it is possible to devise custom shapes that allow only certain colors to be transmitted. Even better, more complex surface shapes can generate unusual light effects including iridescence, for example.”
Unbeknownst to me, this has been known for many years. However, the problem in using the technique was that the generation of appropriate surfaces was a very complex matter. Now, that’s changed as the researchers have developed an easier way to do so. They explain:
“The common approach for creating suitable structures for structural colorizations is either to replicate designs from nature or to emphasize a certain physical effect. In both cases, the structures usually have only a few degrees of freedom and the corresponding (often continuous) parameter space can be efficiently searched either by simulation or fabrication.
An alternative approach is to parameterize very general structures with many degrees of freedom and to employ some computational optimization to find suitable parameters that realize a desired colorization. The most general approach of topology optimization allows the treatment of complex physical effects, e.g., band-gap design of photonic crystals or – similar to our work – structural color, but generally yield designs in the form of complex patterns that are not easily amendable to fabrication.
We employ an intermediate approach, where we use a structure parameterization that constrains the design space to a fabricable subspace.”
The researchers, having generated arbitrary patterns for generating colors, embarked on 3D printing these structures. Their approach was to use multiphoton lithography, an unusual microscopic 3D printing technology that involves use of a defocused laser that is brought to focus in the midst of a gel of photopolymer. The focal point provides sufficient energy to enable the photo polymerization reaction, only at a minuscule point proportional to the power of the laser and size of the focal area. This 3D printing technique is used to produce all sorts of very tiny structures for a variety of industrial applications.
It’s an ideal 3D printing method for this experiment. But did it work? They explain:
“As a result of the optimization problem in Equation (6), we obtain a nanostructure that realizes a given colorization objective. The description of the structure already well-suited for our fabrication method and only minor post-processing is required.”
![3D printed color cell examples, where desired colors are transmitted [Source: ACM]](https://fabbaloo.com/wp-content/uploads/2020/05/3dp-color-cell-examples_result_img_5eb0a8347d0aa.jpg)
They were able to generate a wide variety of structures that enable a considerable number of color combinations.
Now it gets even more interesting. By being able to generate such patterns by software, you can create far more complex color surfaces. For example, a color gradient could be devised. Mixes of colors are possible. They say that by restricting the viewing angle it is possible to create arbitrary color filters.
And these structures are physical, so that they do not fade over time due to chemical effects. However, their performance is dependent on their structure, so if you were brush against them, the structure would be disturbed and colors would be lost. I suspect it may be possible to coat them with a protective clear layer, however.
I’m wondering how this color 3D printing technology could be applied, and have many ideas. One of them, obviously, would be to be included in future 3D printed fashions and shoes. Imagine being able to request all types of radical color patterns from a software tool and obtaining the physical result a short while later.
This research adds another piece to the jigsaw puzzle of future manufacturing. While initial uses of the approach are likely to be quite pricey and thus constrained to only certain expensive applications, the cost will over time drop and we could begin to see 3D printed colors in more everyday objects in the future.
“Computational design of nanostructural color for additive manufacturing” was authored by Thomas Auzinger, Wolfgang Heidrich and Bernd Bickel.
Via ACM
A research thesis details the incredibly complex world of volumetric 3D printing. We review the highlights.